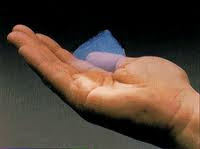
CROATIAN CENTER of RENEWABLE ENERGY SOURCES
What is Aerogel?
A general introduction to aerogels as a class of materials along with an overview of the different properties different types of aerogels can take on.
How is Aerogel Made?
An overview of how aerogels are made and insights into the structure of aerogels from a nano perspective.
Properties of Aerogels
Interactive tables of materials properties for different types of aerogels, nanofoams, and common materials for comparison.
Aerogels are a diverse class of porous, solid materials that exhibit an uncanny array of extreme materials properties. Most notably aerogels are known for their extreme low densities (which range from 0.0011 to ~0.5 g cm-3). In fact, the lowest density solid materials that have ever been produced are all aerogels, including a silica aerogel that as produced was only three times heavier than air, and could be made lighter than air by evacuating the air out of its pores. That said, aerogels usually have densities of 0.020 g cm-3 or higher (about 15 times heavier than air). But even at those densities, it would take 150 brick-sized pieces of aerogel to weigh as much as a single gallon of water! And if Michaelangelo’s David were made out of an aerogel with a density of 0.020 g cm-3, it would only weigh about 4 pounds (2 kg)! Typically aerogels are 95-99% air (or other gas) in volume, with the lowest-density aerogel ever produced being 99.98% air in volume.
Essentially an aerogel is the dry, low-density, porous, solid framework of a gel (the part of a gel that gives the gel its solid-like cohesiveness) isolated in-tact from the gel’s liquid component (the part that makes up most of the volume of the gel). Aerogels are open-porous (that is, the gas in the aerogel is not trapped inside solid pockets) and have pores in the range of <1 to 100 nanometers (billionths of a meter) in diameter and usually <20 nm.
Aerogels are dry materials (unlike “regular” gels you might think of, which are usually wet like gelatin dessert). The word aerogel refers to the fact that aerogels are derived from gels–effectively the solid structure of a wet gel, only with a gas or vacuum in its pores instead of liquid.
Technical Definition
By definition,
An aerogel is an open-celled, mesoporous, solid foam that is composed of a network of interconnected nanostructures and that exhibits a porosity (non-solid volume) of no less than 50%.
The term “mesoporous” refers to a material that contains pores ranging from 2 to 50 nm in diameter.
Generally speaking, most of the pores in an aerogel fall within this size range. In practice, most aerogels exhibit somewhere between 90 to 99.8+% porosity and also contain a significant amount of microporosity (pores less than 2 nm in diameter)
Special Properties of Aerogels
Many aerogels boast a combination of impressive materials properties that no other materials possess simultaneously. Specific formulations of aerogels hold records for the lowest bulk density of any known material (as low as 0.0011 g cm-3), the lowest mean free path of diffusion of any solid material, the highest specific surface area of any monolithic (non-powder) material (up to 3200 m2 g-1), the lowest dielectric constant of any solid material, and the slowest speed of sound through any solid material. It is important to note that not all aerogels have record properties (in fact most don’t, although they may have very good values for many properties)!
By tailoring the production process, many of the properties of an aerogel can be adjusted. Bulk density is a good example of this, adjusted simply by making a more or less concentrated precursor gel. The thermal conductivity of an aerogel can be also be adjusted this way, since thermal conductivity is related to density. Typically, aerogels exhibit bulk densities ranging from 0.5 to 0.01 g cm-3 and surface areas ranging from 100 to 1000 m2 g-1, depending of course on the composition of the aerogel and the density of the precursor gel used to make the aerogel. Other properties such as transparency, color, mechanical strength, and susceptibility to water depend primarily on the composition of the aerogel.
For example, silica aerogels, which are the most widely researched type of aerogel (and the type people typically see in photographs), are usually transparent with a characteristic blue cast due to Rayleigh scattering of the short wavelengths of light off of nanoparticles that make up the aerogel’s framework. Carbon aerogels, on the other hand, are totally opaque and black. Furthermore, iron oxide aerogels are just barely translucent and can be either rust-colored or yellow. As another example, low-density (<0.1 g cm-3) inorganic aerogels are both excellent thermal insulators and excellent dielectric materials (electrical insulators), whereas most carbon aerogels are both good thermal insulators and electrical conductors. Thus it can be seen that by adjusting processing parameters and exploring new compositions, we can make materials with a versatile range of properties and abilities.
Aerogel vs. Silica Aerogel
The term aerogel when used by itself is frequently used to refer specifically to silica aerogels like the blue one shown in the first picture above (although this is like saying “plastic” and specifically meaning, say, polyethylene, despite the fact that there are many other types of plastic such as polypropylene, acrylic, Teflon®, Nylon®, etc.). In general, the aerogel of a substance is chemically similar to the bulk form of that substance. This said, due to their low densities and length-scale effects arising from having nanostructured features, aerogels often exhibit many dramatically enhanced materials properties over the non-aerogel form of the same substance (for example, significant increases in surface area and catalytic activity), while frequently also exhibiting reductions in other materials properties (such as mechanical strength).
The Dire Consequences of Evaporatively Drying a Gel
Now, if you’ve ever left Jell-O uneaten and uncovered in the refrigerator for a long while (on the order of a week or so), you may have observed the gel shrinks gradually. This occurs when the liquid trapped in the gel evaporates from the gel’s surface. As molecules of liquid escape into the air, the surrounding liquid molecules are pulled together by capillary action and tug on the framework of the gel. Continued evaporation results in collapse of the framework of the gel, forming a dense, hard substance with less than 10% of the volume of the original gel. This is called xerogel (pronounced zeroGEL). In fact, 1980′s-style hard contact lenses used to be manufactured by drying silica gels into lens-shaped silica xerogels.
Aerogel is the solid framework of a gel isolated from its liquid component, prepared in such a way as to preserve the framework’s pore structure (or at least most of it). In other words, aerogel is what would be left over if you could remove the liquid from a gel without causing it to shrink. This is most effectively done through a special technique called supercritical drying (although as you will see below, there are other ways to make aerogel as well).
The Answer: Supercritical Drying
In general, supercritical drying is used when liquid needs to be removed from a sample that would be damaged by evaporative or other drying techniques. Biological specimens, for example, are often preserved through supercritical drying.
Supercritical drying is a clever technique by which we can pull the rug out from under capillary action (so to speak). As mentioned earlier, capillary action induced by liquid evaporating from a gel’s pores causes the gel to shrink. So what if there were some way to avoid capillary forces to begin with? This is where supercritical drying comes in.
All pure substances (that won’t decompose) have what’s called a critical point–a specific and characteristic pressure and temperature at which the distinction between liquid and gas disappears. For most substances, the critical point lies at a fairly high pressure (>70 atmospheres) and temperature (>400°F). At the critical point, the liquid and vapor phases of a substance merge into a single phase that exhibits the behavior of a gas (in that it expands to fill the volume of its container and can be compressed) but simultaneously possesses the density and thermal conductivity of a liquid. This phase is called a supercritical fluid.
Say we have a sealed container containing a liquid below its critical point inside and equipped with a pressure gauge on top. In fact, a certain amount of liquid will evaporate in the container until the vapor pressure of the liquid is reached in the container, after which no more liquid will evaporate and the gauge will read a corresponding stable pressure. Now if we heat this container, we will notice the pressure in the container increases, since the vapor pressure of a liquid increases with increasing temperature. As the critical point draws near, the pressure in the container squeezes molecules in the vapor close enough together that the vapor becomes almost as dense as a liquid. At the same time, the temperature in the container gets high enough that the kinetic energy of the molecules in the liquid overwhelms the attractive forces that hold them together as a liquid. In short, as the pressure and temperature in the container get closer to the critical point, the liquid phase becomes more gas-like and the vapor phase more liquid-like. Finally, the critical point is reached and the meniscus dividing the two phases blurs away, resulting in a single supercritical phase. As this occurs, the surface tension in the fluid gradually drops to zero, and thus the ability of the fluid to exert capillary stress does too.
Aerogelification
In the case of making aerogels, a gel is placed in a pressure vessel under a volume of the same liquid held within its pores (lets say ethanol for example). The pressure vessel is then slowly heated to the liquid’s critical temperature. As this happens, the vapor pressure of the liquid increases, causing the pressure in the vessel to increase and approach the critical pressure of the liquid. The critical point is then surpassed, gently transforming the liquid in the gel (as well as the liquid and vapor surrounding the gel) into a supercritical fluid. Once this happens, the ability of the fluid in the gel to exert capillary stress on the gel’s solid framework structure of the gel has decreased to zero.
With supercritical fluid now present throughout the entire vessel and permeating the pores of the gel, the fluid in the gel can be removed. This is done by partially depressurizing the vessel, but not so much as to cause the pressure in the vessel to drop below the critical pressure. The temperature of the vessel must also remain above the critical temperature during this step. The goal is to remove enough fluid from the vessel while the fluid is still supercritical so that when the vessel is fully depressurized/cooled down and drops below the fluid’s critical point, there will simply not be enough substance left in the vessel left for liquid to recondense. This might require several cycles of heating (and thus pressurizing) followed by depressurization (again all done above the critical point). Once enough fluid has been removed from the vessel, the vessel is slowly depressurized and cooled back to ambient conditions. As this happens, the fluid in the vessel passes back through the critical point, but since much of the fluid has been removed and the temperature is still elevated as the vessel depressurizes, the fluid reverts to a gas phase instead of a liquid phase. What was liquid in the gel has been converted into a gas without capillary stress every arising, and an aerogel is left behind.
It is important to note, however, that most of the liquids used in the preparation of gels are organic solvents such as methanol, ethanol, acetone, and acetonitrile, and such liquids are potentially dangerous at the temperatures and pressures required to make them supercritical. To make the aerogelification process less dangerous, the liquid component of a gel can be exchanged with a non-flammable solvent that mixes well with organic solvents–liquid carbon dioxide.
The Start of an Aerogel: A Gel
Aerogels start their life out as a gel, physically similar to Jell-O®. A gel is a colloidal system in which a nanostructured network of interconnected particles spans the volume of a liquid medium. Gels have some properties like liquids, such as density, and some properties like solids, such as a fixed shape. In the case of Jell-O, this network of particles is composed of proteins and spans the volume of some sort of fruit juice. A gel is structurally similar to a wet kitchen sponge, only with pores a thousand to a million times smaller. Because a gel’s pores are so small, the capillary forces exerted by the liquid are strong enough to hold it inside the gel and prevent the liquid from simply flowing out. It’s important to remember that gelatin isn’t the only type of gel–in fact, chemists can prepare gels with backbones composed of many organic and inorganic substances and many liquid interiors.
Once a gel is prepared, it must be purified prior to further processing. This is because the chemical reactions that result in the formation of a gel leave behind impurities throughout the gel’s liquid interior that interfere with the drying processes used to prepare aerogel (as described below). Purification is done by simply soaking the gel under a pure solvent (depending on the gel this could be acetone, ethanol, acetonitrile, etc.), allowing impurities to diffuse out and pure solvent to diffuse in. The solvent in which the gel is soaked is typically exchanged with fresh solvent multiple times over the course several days. Depending on the volume and geometry of the gel, diffusive processes can take any where from hours to weeks. A ice-cube size sample can usually be purified in 1 or 2 days.
All info about AEROGEL at: http://www.aerogel.org/
Will Walker
More info at http://solarserdar.blogspot.com.
CROATIAN CENTER of RENEWABLE ENERGY SOURCES (CCRES)
This comment has been removed by a blog administrator.
ReplyDelete